Non-invasive PET imaging is playing an increasingly important role in cancer diagnosis and staging as it allows sensitive and deep-tissue localization of tumors and metastases. In comparison to MRI or SPECT, PET combines very high sensitivity with dynamic spatio-temporal resolution to examine bio-distribution and clearance. In a study of 3,779 Canadian patients, PET imaging resulted in treatment modifications in 83% of cases. Clinically, PET is now used in treatment selection criteria to benefit patients who will respond to targeted therapies and for confirming treatment response. FDA initiatives now promote co-development of molecular diagnostics to improve drug development efficiency and patient outcomes; nearly two-thirds of FDA-granted therapy designations include a companion diagnostic. Canada has recognized the importance of PET by investing in cyclotron infrastructure for tracer production in every province. In the past 20 years, cancer subtypes are increasingly distinguished by peptides and other large molecules designed to identify target-based diagnostics and therapeutics. Labeled peptides distinguish pathologically distinct cell types by recognizing specific targets, which is impossible to do with FDG. Radiolabeled peptides, which show superior tissue penetration compared to antibodies as reviewed, are now used to treat prostate cancer (PCa) and neuroendocrine cancers (NETs).
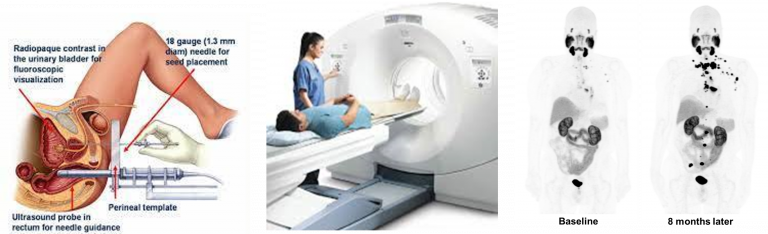
Left: fine-needle biopsy to first confirm cancer, Middle: patient undergoing PET scan; Right: patient with initial metastatic prostate cancer and same patient with detectable metastatic spread indicating poor response to current chemotherapy.
Peptides, which are increasingly used as drug and as targeting agents are excellent scaffolds for developing the next generation of precision agents for radiotheranostic development. Of the isotopes available for PET imaging, radioactive 18F-fluoride is the only scalable isotope available for imaging millions of cancer patients. Yet its well-known lack of reactivity in water has challenged the mass-development of radiopharmaceuticals.
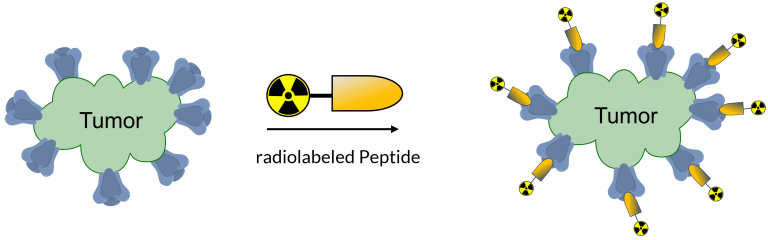
Concept of targeted precision radiotheranostics where a radiolabeled biologic drug e.g. peptide is radiolabeled for imaging and/or therapy.
Thinking out of the box, one of our first skunkworks projects was to invent, disclose and capitalize a fully aqueous, user-friendly, one-step, last-step radiofluorination methodology for labeling peptides and then clinically translate this discovery. Hence, instead of fluorinating a carbon acceptor in water (difficult), we looked left on the periodic table to boron for capturing 18F in water. With no precedence for this, we developed and patented a boron-tag method that enables the synthesis of precursor peptidic boron conjugates that are radiofluorinated in one-step.
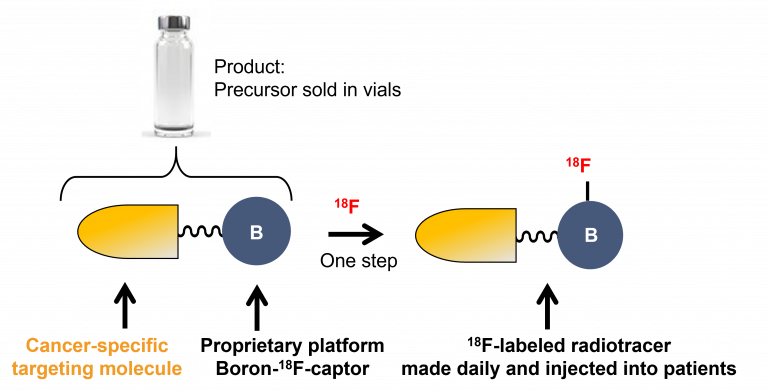
Concept of a boron-assisted one-step radiofluorination
Synthesizing novel boronates including new ones that have never been prepared to understand the free energy relationships for finding a “sweet spot” of kinetic stability at physiological pH while enabling labeling by isotope exchange at pH 3, we reduced this approach to practice as we developed kit-like methodology for radiofluorination.
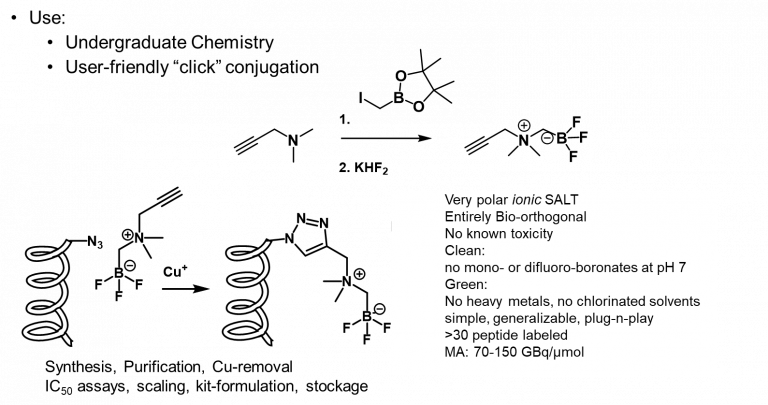
User-friendly kit-like assembly of radiotracers: undergraduate SN2 reaction, trifluoroborate synthesis and easy “click” conjugation to a peptide of choice. Radiofluorination occurs via isotope exchange.
While we have labeled over 30 peptides with this method, we worked to translate an octreotate-BF3 conjugate to first-in-human studies with our collaborators at BC Cancer.

Left: comparative structures of 18F-AMBF3-TATE and 68Ga-DOTA-TATE with corresponding phantoms showing higher resolution for 18F; Middle l/r: image in mouse of 18F-AMBF3-TATE and tumor:non-tumor contrast ratios compared to 68Ga-DOTA-TATE; right: first in human PET image.
While we are always questing for new proprietary organoboronic acids that will be useful for radiofluorination and patentable, we have begun to extend this approach to therapeutic applications in creating first-in-class “true” theranostics provide for theranostic pairs of cold-hot/hot-cold isotopic loading, also known as radiohybrids where a radiometal chelator is appended to enable metallation with a therapeutic radiometal e.g. lutetium. These new theranostics are isotopologs that are chemically identical, only differing by their isotopic loading; hence we will use 18F/175Lu for imaging and 19F/177Lu for therapy. This concept shown below builds on 40 years of radiotheranostic development but instead of using two different radiometals that confer different biological properties, this new radiohybrid approach stands to revolutionize the way radiotracers will be conceived and deleloped.
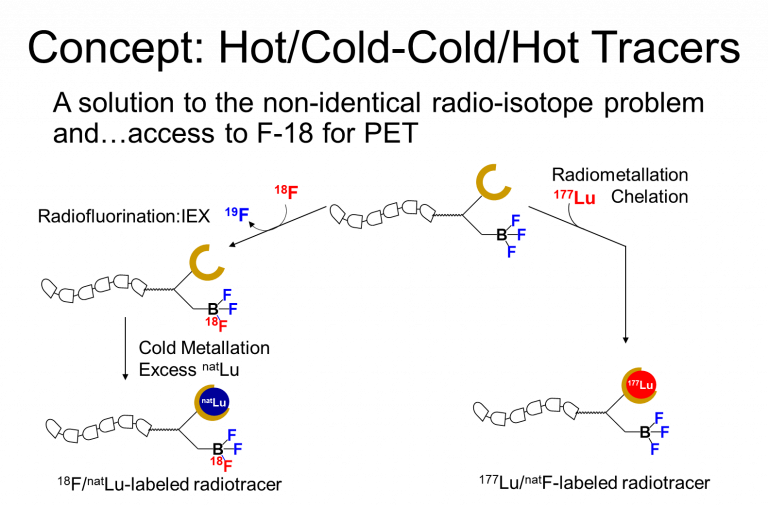
radiohybrid theranostic development.